Caltech researchers take a direct image of magic-angle twisted graphene sheets
Just over a year after researchers at MIT stunned the physics world with the discovery of the "magic angle" for stacked sheets of graphene, researchers at Caltech have directly observed and studied this material using a scanning tunneling microscope that can image electronic properties at atomic-length scales.
Understanding the "magic angle"—a specific orientation between the stacked graphene that yields special electric properties—could pave the way to realizing the dream of room-temperature superconductors, which could transmit enormous electric currents while producing zero heat.
But first: what is the magic angle? Say you take two sheets of graphene—single-atom thick lattices of carbon atoms—and lay one atop the other to create a bilayer material, then twist one of the sheets of graphene to shift their orientation to one another. As the orientation shifts, the electronic properties of the bilayer material will change with it. In early 2018, researchers at MIT discovered that, at a certain orientation (about 1.1 degrees of relative twist), the bilayer material, surprisingly, becomes superconducting and moreover, the superconducting properties can be controlled with the electric fields. Their discovery launched a new field of research into magic angle-oriented graphene, known as "twistronics."
Engineers and physicists at Caltech have built upon that discovery by generating an image of the atomic structure and electronic properties of magic angle-twisted graphene, yielding new insight into the phenomenon by offering a more direct way of studying it. A paper on their work was published in the journal Nature Physics on August 5.
"This pulls back the shroud on twistronics," says Caltech's Stevan Nadj-Perge, corresponding author of the paper and assistant professor of applied physics and materials science in the Division of Engineering and Applied Science.
Research on the magic angle requires an extreme level of precision to get the two sheets of graphene aligned at just the right angle. Old techniques for doing so necessitated embedding the graphene in an insulating material, which had the unfortunate side effect of preventing direct study of the sample. Instead, researchers had to use indirect methods of probing the graphene sample—for example, by taking measurements of how electrons flow through it. Nadj-Perge and his colleagues developed a new method of creating samples of magic angle-twisted graphene that can be used to align the two sheets of graphene very precisely while leaving it exposed for direct observation.
Using this technique, the researchers could learn more about the electronic properties of the material at the magic angle as well as study how these properties change as the twist angle moves away from the magic value. Their work provided several key insights that will guide future theoretical modeling and experiments, including the observation that the electronic correlation plays an important role near the charge-neutrality point—the angle at which the bilayer is electronically neutral.
"Previously, it was thought that correlation effects do not play a major role in charge neutrality," Nadj-Perge says. "Closer, more detailed examination of samples like this could help us to explain why the exotic electronic effects near the magic angle exist. Once we know that, we could help pave the way for useful applications of it, perhaps even leading to room-temperature superconductivity one day."
The paper is titled "Electronic correlations in twisted bilayer graphene near the magic angle." Co-authors of the paper include Gil Refael, Taylor W. Lawrence Professor of Theoretical Physics; Jason Alicea, professor of theoretical physics; Caltech graduate students Youngjoon Choi, Harpreet Arora, Robert Polski, and Yiran Zhang; Caltech postdoctoral scholars Jeannette Kemmer, Yang Peng, Alex Thomson, Hechen Ren; as well as Felix von Oppen of Freie Universität Berlin in Germany; and Kenji Watanabe and Takashi Taniguchi of the National Institute for Materials Science in Japan. This research was funded by the National Science Foundation, the Kavli Nanoscience Institute, the Institute for Quantum Information and Matter at Caltech, the Walter Burke Institute for Theoretical Physics at Caltech, the Deutsche Forschungsgemeinschaft, the Kwanjeong Educational Foundation, and the Transregional Collaborative Research Center 183.
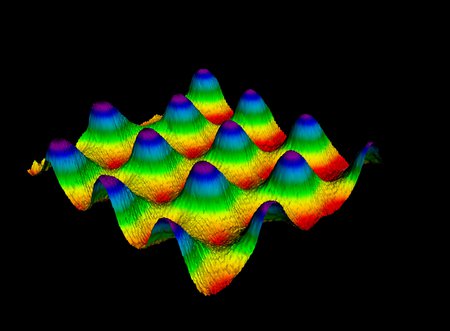
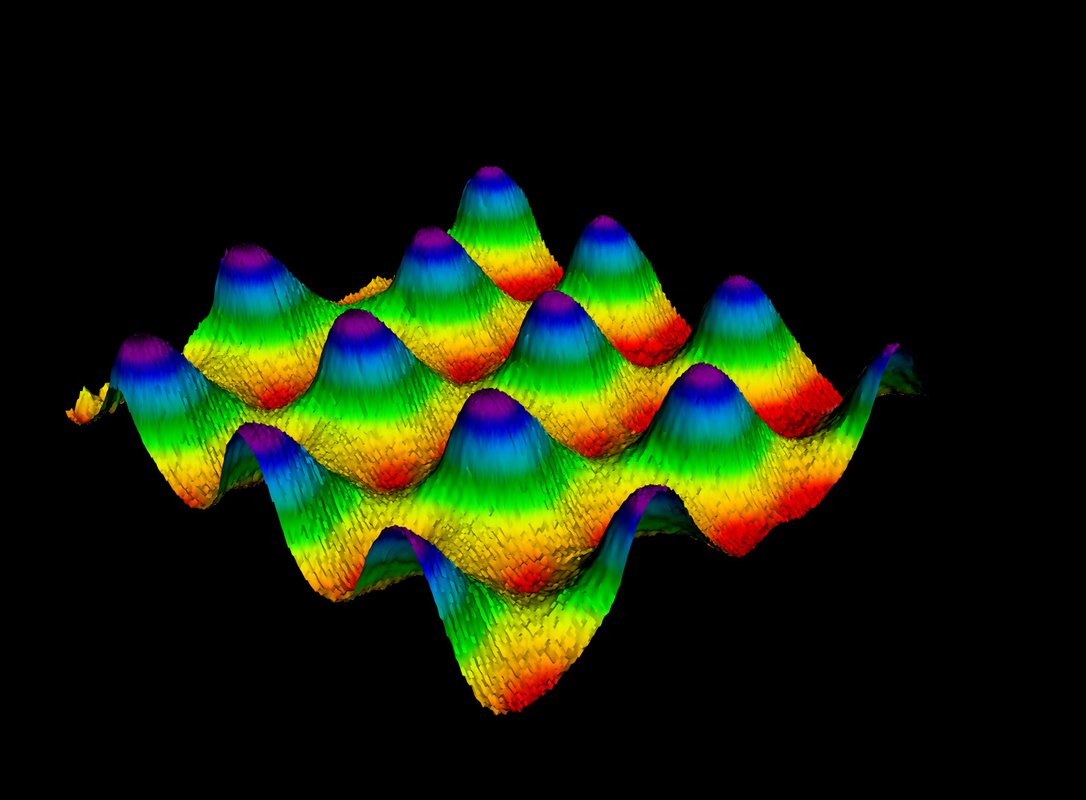